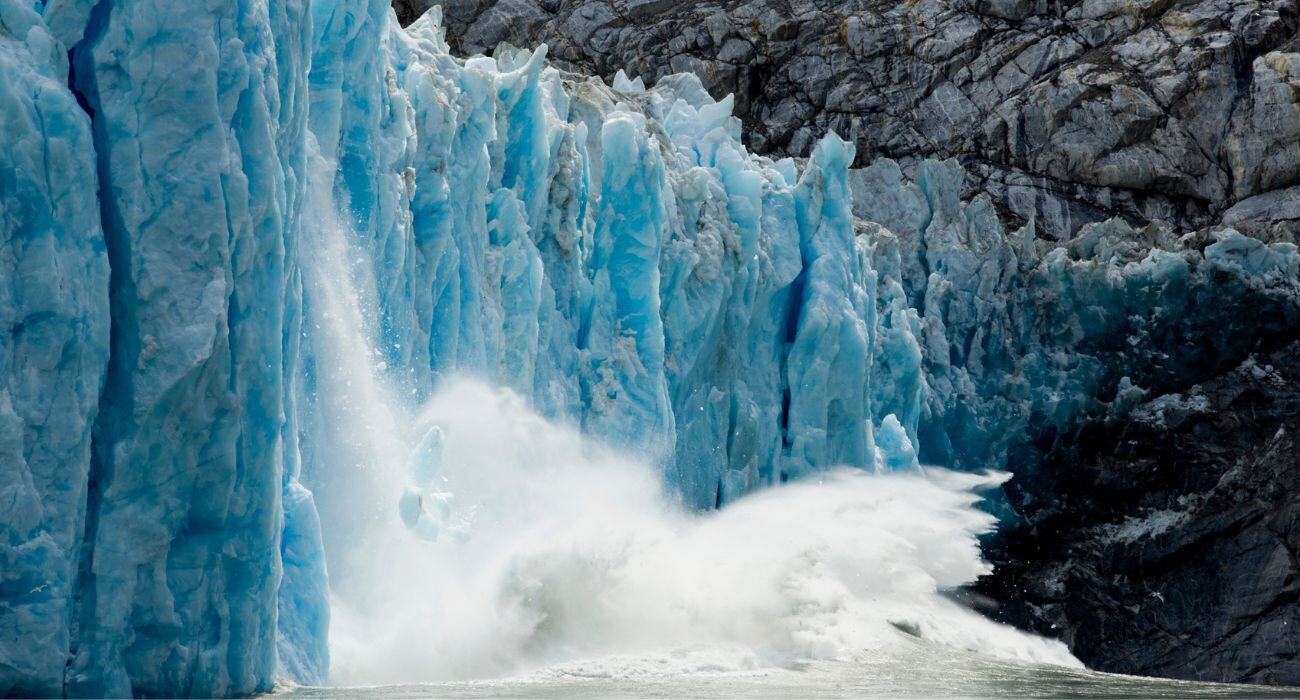
From technological leaps to political revolutions, many transitions happen not in slow and predictable steps, but as abrupt and often irreversible shifts. This is also true for climate change, where tipping points in our oceans, ice sheets, and beyond have the potential to cause irreversible damage to ecosystems, economies, and societies.
Understanding tipping points is crucial for anticipating future physical, transition, and systemic climate risks. This article explores the origins of tipping points, how they function, and their profound implications for climate policy and risk management.
What Is a Tipping Point?
The idea of the tipping point was first applied to climate change in the early 2000s, but the concept is at least 50 years older. It can be found — sometimes as a metaphor and sometimes as a technical concept — across political science, sociology, economics, ecology, and more recently, earth system science.
Tom Strachan
In every application, a tipping point is “a critical threshold beyond which a system reorganises, often abruptly and/or irreversibly” (IPCC). This results in a definitive shift from one stable state to another. This movement can be caused by disproportionately small and/or gradual initial forcing.
The main mechanism behind tipping points is the feedback loop. A feedback loop can be positive or negative.
- In a negative feedback loop, an action has a consequence that dampens the effect of the initial action. For example, as temperatures increase, more water evaporates from the ocean, rivers and lakes, forming clouds that reflect sunlight back into space. This reduces the amount of solar radiation that reaches Earth's surface, thus lowering surface temperatures. Negative feedback loops reduce the effect of change and help a system to maintain equilibrium.
- In a positive feedback loop, an action has a consequence that amplifies the effect of the initial action. For example, as temperatures increase, more polar ice melts. This means that less sunlight is reflected back into space by polar ice (a process known as “albedo”) which causes more warming and further ice melt (see Figure 1). Positive feedback loops increase the effect of change and cause a system to move further away from equilibrium.
Figure 1: The Ice-Albedo Feedback
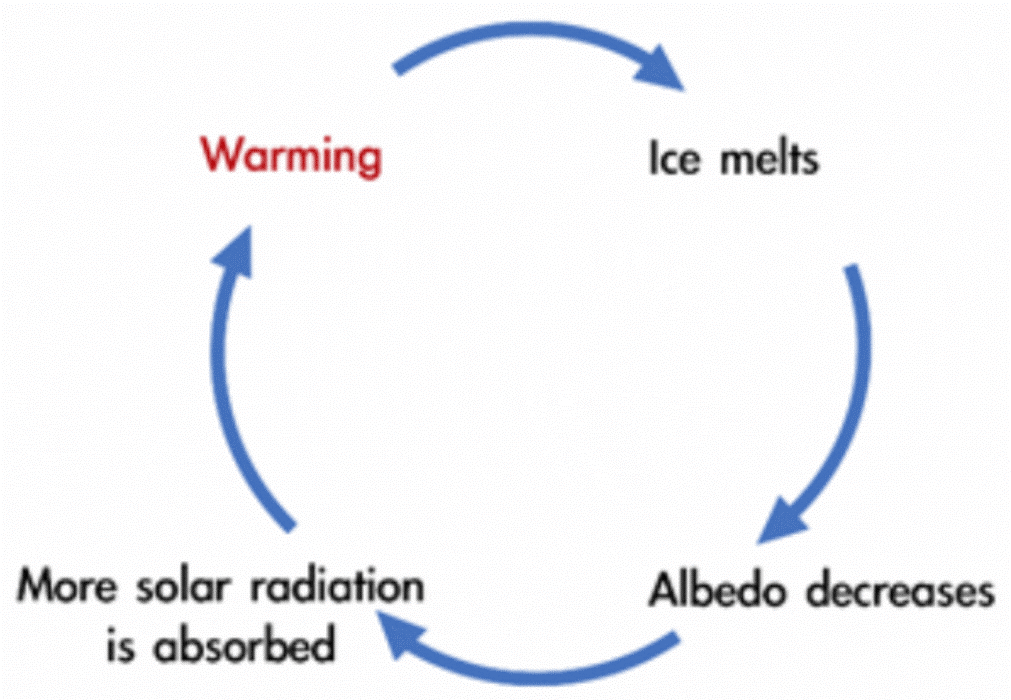
Diagram credit to NASA.
Positive and negative feedback loops often work together to allow a system to undergo change, achieve a desired outcome, and then return to equilibrium. However, some systems can have a particular kind of positive feedback loop, where the amplification effect becomes so powerful that the system essentially runs away under its own momentum — this moment of ‘running away’ is the tipping point. This forces the system into a non-linear transition from one stable state to another. A system or the part of a system where this tipping point can occur is known as the tipping element.
Tipping Points and Climate Change
Tipping points caused by atmospheric warming, or “climate tipping points,” have gained significant attention in recent years. This began around 2007 when a synthesis of early research on possible tipping points in the climate system was featured in the Intergovernmental Panel on Climate Change’s (IPCC) Fourth Assessment Report, highlighting them as a potentially dangerous gap in current understandings of physical climate change.
Another key publication, also from 2007, was “Tipping the scales” by earth system scientists Tim Lenton and Hans Joachim Schellnhuber, which highlighted the implications of tipping points for climate policy.
To paraphrase Lenton and Schellnhuber, climate change is typically understood as a relatively smooth and linear process, where change is proportional to warming, and warming is proportional to emissions. However, climate tipping points challenge this thinking because they are distinctly non-linear. In other words, they can cause change that is disproportional to warming, resulting in “high-casualty and high-cost impacts.” Due to the potential for severe consequences, the threat of tipping points requires a combination of strong mitigation policy and close monitoring of tipping elements for early signs of change.
The current scientific consensus on climate tipping points is summarized by the IPCC:
“The likelihood and impacts of abrupt and/or irreversible changes in the climate system, including changes triggered when tipping points are reached, increase with further global warming (high confidence) … The probability of low-likelihood outcomes associated with potentially very large impacts increases with higher global warming levels (high confidence).”
However, the current policy response to tipping points lags significantly behind scientific understanding of them as major risks. Global Tipping Points, a coalition of climate tipping point experts, described the inadequacy of current policy:
“Stopping these threats is possible but requires urgent global action. Global governance is currently inadequate to minimise tipping point threats and to do so equitably. Governance is needed across multiple scales to address the different drivers, potentially rapid changes, and diverse, often irreversible, impacts of tipping points.”
Notwithstanding this, not all tipping points are considered policy relevant. For example, the global average temperature increase required to trigger a given tipping point might be too great for it to occur within the next 100 years — even in a worst-case emissions scenario —– and is therefore seen as superfluous to current climate policy concerns.
Known Climate Tipping Points
Tipping points have been identified in virtually all parts of the climate system. They vary in their:
- Critical warming threshold – the amount of warming (+◦C above the pre-industrial baseline) required to trigger the tipping point;
- Transition timescale – the length of time between the triggering of the tipping point and its full impact(s);
- Reversibility – the degree to which the tipping element can(not) return to its previous stable state.
- Impacts – the geographical scope and severity of the tipping point’s impacts;
For most tipping points, there is still significant uncertainty around all four of these variables. Table 1 lists parts of earth systems with a medium or higher confidence of being a tipping element, which also have a clear critical warming threshold according to latest available research. For a single tipping element, best estimates of critical warming thresholds can vary from current or near-current warming to 6◦C or more. Similarly, transition timescales can range from just over a decade to 300 years.
This uncertainty makes climate tipping points especially dangerous and hard to manage.
Table 1: Parts of Earth Systems with a Medium or Higher Confidence of Being a Tipping Element Which Also Have a Clear Critical Warming Threshold
1Global Tipping Points Report (2023)
2Tipping elements in the Earth's climate system (2008)
3Exceeding 1.5°C global warming could trigger multiple climate tipping points (2022)
4Reduced CO2 uptake and growing nutrient sequestration from slowing overturning circulation (2022)
Parting Thoughts
Climate tipping points force us to change how we think about climate risk, especially about the non-linearity of future physical climate change. This is a particular challenge for risk management, which tends to be grounded in historical data. Moreover, tipping points and their impacts tend to be absent from most climate scenarios, including those of the IPCC, making them even harder to anticipate and mitigate.
Every day of continued emissions increases the chance of triggering one of many abrupt, irreversible and extremely costly events. In addition, there is growing evidence that climate tipping points can cascade, with one event triggering another in a catastrophic domino effect. This cascade can also move outside the climate system, triggering tipping points in populations (e.g., forced mass migrations) and financial systems (e.g., Minsky moments).
From a policy perspective, prevention of climate tipping points through rapid mitigation of greenhouse gas emissions must be the top priority, in conjunction with adaptation for unavoidable tipping point impacts.
A more recent idea for achieving these policy goals is utilizing positive social tipping points: positive feedback loops in society that can accelerate the transformative changes needed for the transition to net zero. With targeted interventions, these positive tipping points can be deliberately triggered, for example to exponentially increase the uptake of renewable energy or to rapidly shift populations towards more climate-friendly diets.
For a deep-dive on climate tipping points, systems theory and the complexity of climate risk, hear from Tim Lenton on the GARP Climate Risk Podcast.
To learn more about how to identify, assess and manage climate and nature-related risks facing your business, consider signing up for our Sustainability & Climate Risk (SCR) Certificate.
--
Tom Strachan is Assistant Vice President at the GARP Risk Institute, specializing in sustainability, climate risk and nature risk management. He holds a BA in Geography from the University of Exeter and a MSc in Environmental Technology from Imperial College London.
Article Updated: February 7, 2025
Topics: Physical Risk, Transition Risk, Climate Risk Management